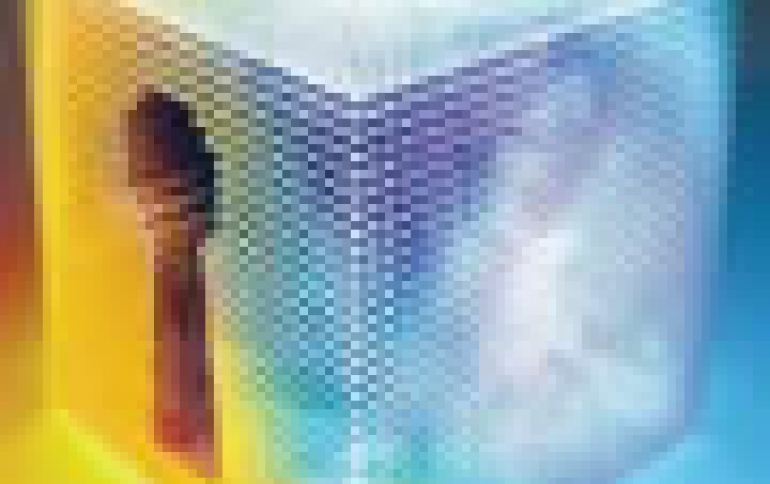
University of Tokyo, Fujitsu and NEC Bring Quantum Cryptography A Step Closer to Practical Implementation
Institute for Nano Quantum Information Electronics, The University of Tokyo, Fujitsu Laboratories Limited, and NEC today announced that they have achieved quantum cryptographic key distribution at a world-record distance of 50 km using transmission from a single-photon emitter.
This result was the product of the three-way collaboration between The University of Tokyo, Fujitsu, and NEC, and represents a milestone in quantum key distribution because it combines a single-photon emitter , which is ideal for optical transmission bands, with a prototype of a practical quantum cryptography system. Experimental success at a practical level with a single-photon emitter brings one step closer the practical use of quantum cryptography in the 1.5 ?m band, which is most desirable for long-distance data transmissions.
A report on this experiment was published today in the online edition of Applied Physics Express (APEX).
This research can be considered the first in the world to bring together work in quantum-dot optical devices with quantum information technology. Future work will focus on making systems using single-photon emitters more efficient, with the goal of practical implementations in 5?10 years. Also being developed is a current-injected 1.5 ?m single-photon emitter, which promises simpler and more compact quantum cryptographic communications systems in the future.
Background and Technological Challenges
With the spread of the Internet, the need for more secure data communications has grown in order to protect commercial transactions, personal information, and other kinds of sensitive data. The security of public-key cryptography (currently the most common method of cryptography) is measured by the computing power needed to break the key, and as computing technology improves, may become vulnerable in the future. With quantum cryptography, a quantum cryptographic key is transmitted one photon at a time, the smallest increment possible. If an eavesdropper is listening in on the transmission line, the basic principles of quantum theory mean that the act of eavesdropping will change the signal in a way that the intended recipient can immediately detect. The ability to detect eavesdropping as a basic consequence of physical laws is quantum cryptography's greatest advantage over previous cryptographic methods and is the focus of intensive R&D efforts around the world.
Ideally, quantum cryptography uses a single-photon emitter that can generate photons one at a time, but most previous experiments in quantum cryptographic key distribution used extremely attenuated laser light as a pseudo single-photon emitter. One of the problems with pseudo single-photon emitters is that a single pulse would emit two or more photons, which meant that eavesdropping might go undetected. Using a single-photon emitter that operated in the 1.5 ?m band would be ideal for transmitting quantum cryptographic signals over long distances, and would have the advantages of greatly simplifying systems and security analyses.
To make quantum cryptographic key distribution using a single-photon emitter a practical reality, one big hurdle has been improving light sources. While there have been some reported experimental successes of using single-photon emitters for quantum key distribution, they were all limited to bands in the range of 0.64?1.3 ?m, which have a maximum transmission distance of 35 km. To achieve longer transmission distances would require a single-photon emitter operating in the 1.5 ?m band, which minimizes optical losses in the fiber for better performance. This type of single-photon emitter, if demonstrated to have the desired performance in a practical system, would represent a major milestone towards making quantum cryptography with single-photon emitters a practical reality.
A report on this experiment was published today in the online edition of Applied Physics Express (APEX).
This research can be considered the first in the world to bring together work in quantum-dot optical devices with quantum information technology. Future work will focus on making systems using single-photon emitters more efficient, with the goal of practical implementations in 5?10 years. Also being developed is a current-injected 1.5 ?m single-photon emitter, which promises simpler and more compact quantum cryptographic communications systems in the future.
Background and Technological Challenges
With the spread of the Internet, the need for more secure data communications has grown in order to protect commercial transactions, personal information, and other kinds of sensitive data. The security of public-key cryptography (currently the most common method of cryptography) is measured by the computing power needed to break the key, and as computing technology improves, may become vulnerable in the future. With quantum cryptography, a quantum cryptographic key is transmitted one photon at a time, the smallest increment possible. If an eavesdropper is listening in on the transmission line, the basic principles of quantum theory mean that the act of eavesdropping will change the signal in a way that the intended recipient can immediately detect. The ability to detect eavesdropping as a basic consequence of physical laws is quantum cryptography's greatest advantage over previous cryptographic methods and is the focus of intensive R&D efforts around the world.
Ideally, quantum cryptography uses a single-photon emitter that can generate photons one at a time, but most previous experiments in quantum cryptographic key distribution used extremely attenuated laser light as a pseudo single-photon emitter. One of the problems with pseudo single-photon emitters is that a single pulse would emit two or more photons, which meant that eavesdropping might go undetected. Using a single-photon emitter that operated in the 1.5 ?m band would be ideal for transmitting quantum cryptographic signals over long distances, and would have the advantages of greatly simplifying systems and security analyses.
To make quantum cryptographic key distribution using a single-photon emitter a practical reality, one big hurdle has been improving light sources. While there have been some reported experimental successes of using single-photon emitters for quantum key distribution, they were all limited to bands in the range of 0.64?1.3 ?m, which have a maximum transmission distance of 35 km. To achieve longer transmission distances would require a single-photon emitter operating in the 1.5 ?m band, which minimizes optical losses in the fiber for better performance. This type of single-photon emitter, if demonstrated to have the desired performance in a practical system, would represent a major milestone towards making quantum cryptography with single-photon emitters a practical reality.