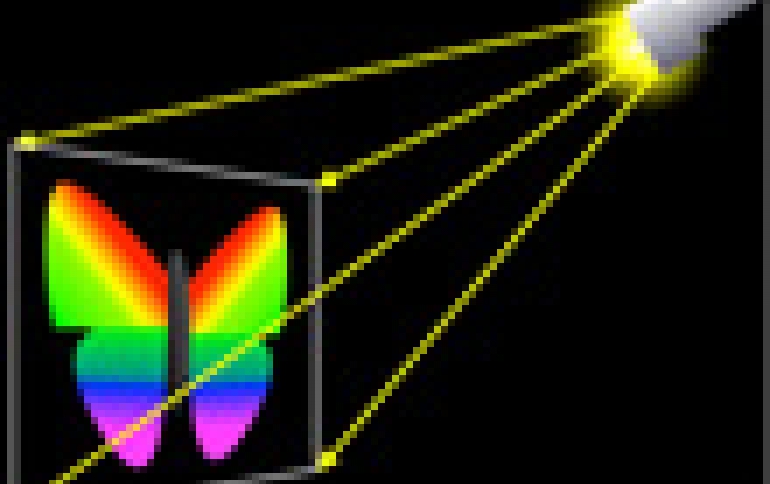
MIT Researchers Unveil Practical New Approach To Holographic Video
Researchers at MIT's Media Lab reported a new approach to generating holograms that could lead to color holographic-video displays that are much cheaper to manufacture than today?s experimental, monochromatic displays.
Using the new technique, Daniel Smalley, a graduate student in the Media Lab, is building a prototype color holographic-video display whose resolution is roughly that of a standard-definition TV and which can update video images 30 times a second, fast enough to produce the illusion of motion. The heart of the display is an optical chip, resembling a microscope slide, that Smalley built, using only MIT facilities, for about $10.
When light strikes an object with an irregular surface, it bounces off at a huge variety of angles, so that different aspects of the object are disclosed when it?s viewed from different perspectives. In a hologram, a beam of light passes through a so-called diffraction fringe, which bends the light so that it, too, emerges at a host of different angles.
One way to produce holographic video is to create diffraction fringes from patterns displayed on an otherwise transparent screen. The problem with that approach is that the pixels of the diffraction pattern have to be as small as the wavelength of the light they?re bending, and most display technologies don?t happily shrink down that much.
Stephen Benton, a Media Lab professor, created one of the first holographic-video displays by adopting a different technique, called acousto-optic modulation, in which precisely engineered sound waves are sent through a piece of transparent material. "The waves basically squeeze and stretch the material, and they change its index of refraction," says Michael Bove, a principal research scientist at the Media Lab and head of its Object-Based Media GroupBove. "So if you shine a laser through it, [the waves] diffract it."
Benton's most sophisticated display , which was built with the help of Bove's group - applied acousto-optic modulation to a crystal of an expensive material called tellurium dioxide. "That was the biggest piece of tellurium dioxide crystal that had ever been grown," Bove says. "And that wasn't TV resolution. So there was a definite scaling problem going on there."
Smalley instead used a much smaller crystal of a material called lithium niobate. Just beneath the surface of the crystal he creates microscopic channels known as waveguides, which confine the light traveling through them. Onto each waveguide, he also deposits a metal electrode, which can produce an acoustic wave.
Each waveguide corresponds to one row of pixels in the final image. In the Mark-II, the tellurium dioxide crystal had to be big enough that the acoustic waves producing the separate lines of the hologram were insulated from each other. In Smalley's chip, on the other hand, the waveguides with their individual electrodes can be packed mere micrometers apart from each other.
Beams of red, green and blue light are sent down each waveguide, and the frequencies of the acoustic wave passing through the crystal determine which colors pass through and which are filtered out. Combining, say, red and blue to produce purple doesn't require a separate waveguide for each color; it just requires a different acoustic-wave pattern.
Bove considers that the most exciting aspect of the new chip. "Until now, if you wanted to make a light modulator for a video projector, or an LCD panel for a TV, or something like that, you had to deal with the red light, the green light and the blue light separately," he says. "If you look closely at an LCD panel, each pixel actually has three little color filters in it. There?s a red subpixel, a green subpixel and a blue subpixel."
Bove's group is currently in talks with the lab?s corporate members about acquiring the chip's technology. As a note, MIT's lab corporate members include LG Electronics and Samsung Electronics.
When light strikes an object with an irregular surface, it bounces off at a huge variety of angles, so that different aspects of the object are disclosed when it?s viewed from different perspectives. In a hologram, a beam of light passes through a so-called diffraction fringe, which bends the light so that it, too, emerges at a host of different angles.
One way to produce holographic video is to create diffraction fringes from patterns displayed on an otherwise transparent screen. The problem with that approach is that the pixels of the diffraction pattern have to be as small as the wavelength of the light they?re bending, and most display technologies don?t happily shrink down that much.
Stephen Benton, a Media Lab professor, created one of the first holographic-video displays by adopting a different technique, called acousto-optic modulation, in which precisely engineered sound waves are sent through a piece of transparent material. "The waves basically squeeze and stretch the material, and they change its index of refraction," says Michael Bove, a principal research scientist at the Media Lab and head of its Object-Based Media GroupBove. "So if you shine a laser through it, [the waves] diffract it."
Benton's most sophisticated display , which was built with the help of Bove's group - applied acousto-optic modulation to a crystal of an expensive material called tellurium dioxide. "That was the biggest piece of tellurium dioxide crystal that had ever been grown," Bove says. "And that wasn't TV resolution. So there was a definite scaling problem going on there."
Smalley instead used a much smaller crystal of a material called lithium niobate. Just beneath the surface of the crystal he creates microscopic channels known as waveguides, which confine the light traveling through them. Onto each waveguide, he also deposits a metal electrode, which can produce an acoustic wave.
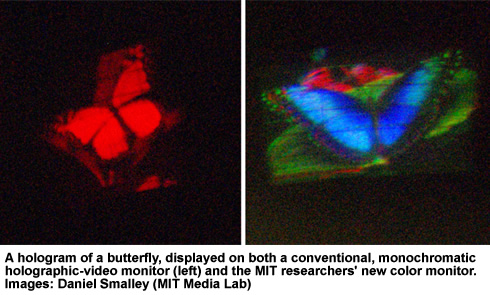
Each waveguide corresponds to one row of pixels in the final image. In the Mark-II, the tellurium dioxide crystal had to be big enough that the acoustic waves producing the separate lines of the hologram were insulated from each other. In Smalley's chip, on the other hand, the waveguides with their individual electrodes can be packed mere micrometers apart from each other.
Beams of red, green and blue light are sent down each waveguide, and the frequencies of the acoustic wave passing through the crystal determine which colors pass through and which are filtered out. Combining, say, red and blue to produce purple doesn't require a separate waveguide for each color; it just requires a different acoustic-wave pattern.
Bove considers that the most exciting aspect of the new chip. "Until now, if you wanted to make a light modulator for a video projector, or an LCD panel for a TV, or something like that, you had to deal with the red light, the green light and the blue light separately," he says. "If you look closely at an LCD panel, each pixel actually has three little color filters in it. There?s a red subpixel, a green subpixel and a blue subpixel."
Bove's group is currently in talks with the lab?s corporate members about acquiring the chip's technology. As a note, MIT's lab corporate members include LG Electronics and Samsung Electronics.