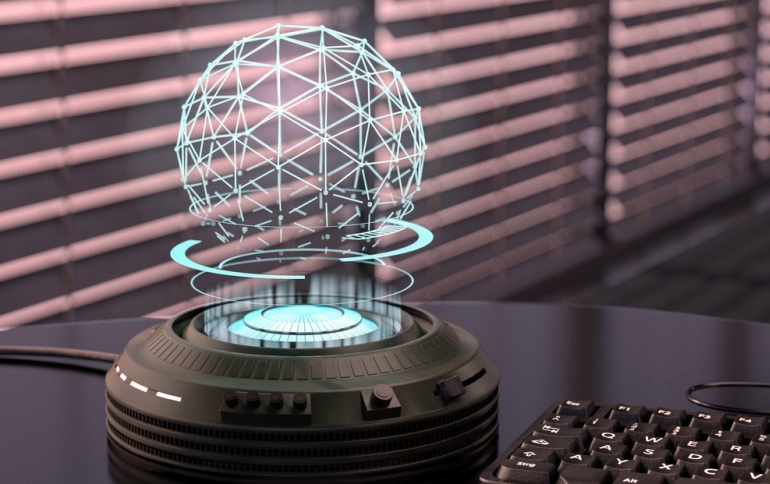
Holographic Technique Could Be Used For Quantum Computation
EPFL physicists have developed a method based on the principles of holograms to capture 3D images of objects beyond the reach of light.
Light is a wave, and is therefore characterized by the phase. Phase specifies the position of a point within the wave cycle and correlates to depth of information, meaning that recording the phase of light scattered by an object can retrieve its full 3D shape, which cannot be obtained with a simple photograph. This is the basis of optical holography, popularized by fancy holograms in sci-fi movies like Star Wars.
But the problem is that the spatial resolution of the photo/hologram is limited by the wavelength of light, around or just-below 1 μm (0.001 mm). That’s fine for macroscopic objects, but it starts to fail when entering the realm of nanotechnology.
Researchers from Fabrizio Carbone’s lab at EPFL have developed a method to see how light behaves on tiniest scale, well beyond wavelength limitations. The researchers used freely propagating electrons as photographic media. Used in their ultrafast electron microscope, the method can encode quantum information in a holographic light pattern trapped in a nanostructure, and is based on an exotic aspect of electron and light interaction.
The scientists used the quantum nature of the electron-light interaction to separate the electron-reference and electron-imaging beams in energy instead of space. This makes it now possible to use light pulses to encrypt information on the electron wave function, which can be mapped with ultra-fast transmission electron microscopy.
The new method can provide two important benefits: First, information on light itself, making it a powerful tool for imaging electromagnetic fields with attosecond and nanometer precision in time and space. Second, the method can be used in quantum computing applications to manipulate the quantum properties of free electrons.
“Conventional holography can extract 3D information by measuring the difference in distance that light travels from different parts of the object,” says Carbone. “But this needs an additional reference beam from a different direction to measure the interference between the two. The concept is the same with electrons, but we can now get higher spatial resolution due to their much shorter wavelength. For example, we were able to record holographic movies of quickly moving objects by using ultrashort electron pulses to form the holograms.”
Beyond quantum computations, the technique has the highest spatial resolution compared to alternatives, and could shift the way we think about light in everyday life. “So far, science and technology have been limited to freely propagating photons, used in macroscopic optical devices,” says Carbone. “Our new technique allows us to see what happens with light at the nanoscale, the first step for miniaturization and integration of light devices onto integrated circuits.”