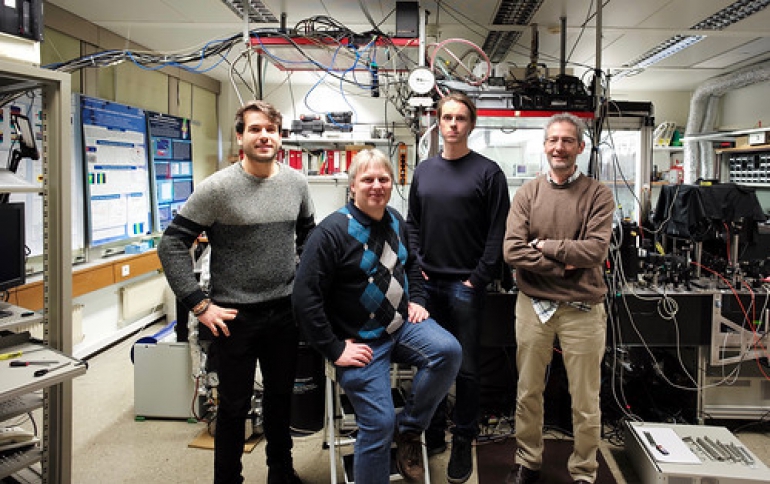
Researchers Build First Ultrafast All-Optical Room Temperature Transistor
IBM researchers have built the first ever cascadable, all-optical transistor capable of operating at room temperature.
The team achieved that by exploiting the material properties of an organic semiconducting polymer. Based on this material, a microcavity was engineered in which an incoming optical signal (a laser beam) can be switched on and off or amplified by another laser beam.
All-optical components that manipulate information with light alone could enable much faster switching and logical operations as well as provide building blocks for new applications like routing “flying qubits” from quantum microwave optical transduction or blind quantum computing. But such all-optical components are very difficult to build. And in fact, efforts to make all-optical computers have been around for about 50 years.
In order to switch or amplify an optical signal with another optical signal, a material that mediates the interaction is needed. It’s just in the quantum nature of light beams that they don’t interact with each other in vacuum. In the new transistor, the mediating part is done by quasi-particles known as exciton-polaritons. They arise in an organic semiconductor (methyl-substituted ladder-type poly- [paraphenylene] or MeLPPP) provided by IBM's partner Prof. Ullrich Scherf from the Wuppertal University. The researchers placed a 35 nanometer-thin layer of MeLPPP between two highly reflective mirrors to form an optical cavity in which exciton-polaritons were produced using a laser. An exciton-polariton consists in the superposition of an exciton (an electron-hole pair) and a photon. That’s why the device falls in the category of organic polariton transistors.
The transistor not only is the first of its kind to work at ambient conditions, it also provides an unprecedented 6500-fold optical signal amplification with a device length of just a few micrometers. That is 330 times higher than the amplification attained by its inorganic counterpart and allows for cascadability, which is a necessary condition to use the transistor for logic gates. In experiments, the device also exhibited the highest net optical gain ever observed for an optical transistor (~10 dB/micrometer).
Furthermore, the transistor features ultrafast switching in the sub-picosecond range, which makes it comparable in terms of multi-terahertz switching speed to some previous all-optical devices with the added advantage that our device doesn’t require cryogenic cooling to operate.
Importantly, the organic polariton transistor gets rid of another limitation present in its inorganic counterparts that is relevant for practical purposes. In inorganic polariton microcavities, the pump laser used to trigger the transistor response must be directed at the device under certain angles only. In the organic device, there’s no specific requirement on the angle of the pump laser which gives much greater flexibility in the geometry of the setting and allows for fiber pig-tailing of the optical device or creating integrated planar circuits with it.
The experiments demonstrate vibron-mediated, dynamic polariton condensation in an organic microcavity at ambient conditions, enabling all-optical polariton amplification, switching at sub-picosecond time scales as well as cascadability and OR and AND logic gate operation. Efficient control over the address state permits reliable switching between ‘low’ and ‘high’ logic levels with ultrafast transient response, while the giant net gain of the structure gives rise to record optical amplification at the micrometer scale.
The developed principles of dynamic polariton condensation in combination with the recently observed frictionless polariton flow in organic microcavities pave the way for on-chip circuitry with ultrafast, all-optical, logic operability. If one could furthermore exploit strong polariton-polariton interactions, where important progress has just been shown earlier this year with inorganic microcavities, such transistors would be able to operate with only a few photons, and thereby drastically lowering the required switching energy to the attojoule regime.
This work was the result of IBM's team at the IBM Research Lab in Zurich together with IBM's partners from the research lab of Prof. Pavlos Lagoudakis at Skolkovo Institute of Science and Technology and Southampton (a collaboration established within the framework of the European Horizon-2020 training network SYNCHRONICS).
Detailed information is available on the cover of the latest edition of the peer-reviewed journal Nature Photonics.